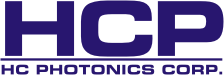
PPLN Guide: Applications
QPM configurations & applications
QPM Configurations & Applications
With its unique capabilities for engineering phase-matching conditions within nonlinear materials, Quasi-Phase Matching (QPM) allows a variety of applications beyond what can be realized by traditional Birefringence-Phase-Matching (BPM). For examples, QPM enables
- Efficient wavelength conversion across full transparency range of selected nonlinear materials under a variety of wave mixing configurations.
- Design and realization of different QPM patterns for diverse applications.
- Conversion efficiency enhancement via QPM based waveguides.
Configurations for efficient wavelength conversion
As a difference from birefringence phase matching (BPM), QPM technique can possibly utilize full transparency ranges of the selected non-linear materials (350 nm~5000 nm for PPLN) and also access to the highest nonlinear d-coefficient (such as for PPLN) to achieve efficient wavelength conversion. Since spatial walk-off issue in general will not occur in QPM configuration, if needed, one can also use the maximum available device length for efficiency optimization (50mm long is common and up to 80mm long is also available for PPLN). This enables efficient wavelength conversion via different wave-mixing configurations for application optimization. Examples of wave mixing configurations (SHG/SFG/DFG/OPA/OPG/OPO) are illustrated in figure 1:
Figure 1: Illustrate wave mixing configuration of (a) SHG, (b) SFG, (c) DFG, (d) OPA, (e) OPG, and (f) OPO.
By designing proper phase matching period, QPM technique will also allow specific type of wave mixing such as Type 0, Type I and Type II for user’s specific application requirement, as illustrated in Figure 2. With QPM, all above types can possibly be realized for selected QPM materials, as one can engineer the phase-matching condition among mixing waves:
(a) In Type 0, two photons with extraordinary polarization will combine to form a one new photon with extraordinary polarization.
(b) In Type I, two photons having ordinary polarization will combine to form a new photon with extraordinary polarization.
(c) In Type II, two photons having orthogonal polarizations will combine to form one photon with ordinary polarization.
Figure 2: Illustrate wave mixing of (a) Type 0, (b) Type I and (c) Type II.
From practical consideration, there are several basic configurations for efficiency optimization:
- Single-pass bulk configuration: by optimizing the input pump power, beam size and interacting length, since the efficiency is (quadratically) proportional to light intensity and interacting length.
- Single-pass waveguide configuration: which reduces the beam size and also eliminates the constraint of beam diffraction for interacting length optimization. However, waveguide coupling, excess waveguide propagation loss, and power handling should also be considered for overall optimization.
- Cavity (such as OPO) configuration: which enhances the effective intensity by confining the light within a resonant cavity structure. However, aligning a precision high-Q cavity with sufficient long-term stability and reliability over the required application condition could be a challenging task.
- Pulse (by ns or ns/ps pulse) configuration: which gives high conversion efficiency due to high peak pulse power and high intensity. High gain OPA or OPG could even possibly be achieved with such a pulse configuration. In general, damage threshold in Q-switched ns configuration and temporal walk-off in ps/fs configuration should be considered. In addition, the converted pulsed output might have a broader spectrum linewidth and might not be suitable for the desired applications.
Optimization for each basic configuration will depend on application requirement and associated practical constraints. Example applications of wave mixing configurations (SHG/SFG/DFG/OPA/OPG/OPO) are highlighted below:
SHG:
(1) >2W CW at 532 nm in single-pass MgO:PPLN bulk by 10~12W pump at 1064 nm
(2) >5W CW at 532 nm in single-pass MgO:PPLT bulk by 20~25W pump at 1064 nm
(3) >28W CW at 780 nm in single-pass MgO:PPLN bulk by ~62W pump at 1560 nm
(4) >1W CW broadly tunable 780 nm in single-pass MgO:PPLN bulk (with fiber-in/fiber-out 1x1 mixer configuration) by 10~12W pump at 1560nm band
SFG:
(1) >3W CW at 632 nm in single-pass MgO:PPLN bulk by SFG of ~7W pump at 1064 nm and 7W pump at 1560 nm
(2) 50 mW CW at 355 nm in single-pass MgO:PPLN bulk by cascaded SHG/DFG of ~2.5W pump at 1064nm
DFG:
>150mW CW at 3346 nm in single-pass MgO:PPLN bulk by DFG of 6.5W pump at 1064 nm and 7W pump at 1560 nm.
OPA:
>38% signal (1580nm)/pump (1064nm) and > 18.4% idler (3257nm)/pump (1064nm), in total >56.8 % of energy conversion efficiency, has been realized in single-pass MgO:PPLN bulk. (test under very week seeding signal and strong Q-switched ns pulsed pump at 1064 nm, with ~4 ns pulse width, repetition rate ~7 kHz, and average power 981mW).
OPG:
OPG threshold (equivalent to OPA gain of >80~90dB) can be reached by peak power less than 10kW in a single-pass MgO:PPLN bulk device. (Test under strong Q-switched ns pulsed pump at 1064 nm, with ~4 ns pulse width, repetition rate ~7 kHz, and average power 400mW).
OPO:
(1) There are numerous published/commercialized broadly tunable CW/pulsed OPO lasers, which can be tuned widely from 1400~1980 nm and 2300~4600nm with 1064 nm pump, or tunable visible (450 – 650 nm) & NIR (900 – 1300 nm) with 532 nm pump. The basic principle behind is based on OPO (or in combination of other SFG configuration), as illustrated above.
(2) One can also put the MgO:PPLN bulk chip inside the laser cavity to further reduce the OPO threshold. >100 mW single-frequency CW signal & idler, with 2~3W of pump diode, has been realized in such an OPO cavity configuration.
QPM patterns for diverse applications
Other advantages of QPM technology are that QPM domain structures are defined by photo-mask patterning process, which provides additional application dimensions unavailable by traditional nonlinear bulk materials. Several other special periodic pattern designs, such as cascaded, fan-out, and other customized configurations are also available for specific applications using the CW, ns pulsed and ultra-short ps/fs pulsed laser pumps. Examples of special QPM structures are illustrated in figure 3-1.
Figure 3-1: Example of various QPM patterns: Uniform, Multiple (Tunable), Cascaded (SHG+SFG/DFG), Chirped (Spectrum Engineering), Fan-out (Continuous tunable) etc.
One can further combine above basic various structures into an array QPM structure for application optimization, as the QPM domain is defined by photo-mask patterning process which is unavailable by traditional nonlinear bulk materials. Examples are shown in figure 3-2 below.
(a) A multiple grating structure with different QPM lengths for ultrafast pulse tuning/or optimization:
(b) A multiple grating structure with different QPM chirping rate for bandwidth tuning and/or optimization:
(c) A multiple grating structure with different QPM fan-out periods for continuous wavelength-fine tuning in the selected wavelength bands:
Figure 3-2: Example of various QPM patterns for application flexibility and optimization.
Conversion efficiency enhancement via QPM waveguides
With the capabilities for engineering phase-matching condition along the desired crystal orientation, QPM also allows the feasibility for fabricating the waveguides in the selected periodically poled materials, which enhance the device efficiency by 2 or 3 orders of magnitude larger than that of the bulk materials and thus enable several applications beyond what can be achieved by the same type of bulk materials. While QPM bulk device offers advantages of higher power and larger aperture applications, QPM waveguide (WG) can be used to further enhance nonlinear wave mixing efficiency by tightly confining the laser intensity over long propagation distance, as shown in figure 4. Certainly, there are pros and cons for selecting bulk configuration vs. waveguide configuration, depending on the practical application requirement (such as required input/output power level) and practical constraints (such as input/output-waveguide coupling). Example applications which can be realized by efficient QPM waveguides are illustrated in figure 5.
Figure 4: Comparison of QPM bulk device and QPM waveguide device
Example applications which can be realized by efficiency QPM waveguides are listed below:
(a) Low-power but high-efficiency wave mixing for visible and mid-Infrared generation.
(b) Efficient parametric amplification (OPA) and parametric generation (OPG)
(c) Entangled photon pairs generation in Quantum Optics
(d) Infrared single photon sensing
(e) Spectrum tailoring for ultrafast or multiple wavelength band conversion
(f) Cascaded Nonlinearity for WDM wavelength band conversion or spectrum inversion for dispersion compensation
(g) Efficient Electro-optics (EO) Modulation
Figure 5: Example applications which can be realized by efficiency QPM waveguides (a)-(g)
(a) Low-power but high-efficiency wave mixing for visible and mid-Infrared generation:
- >100mW CW at 532 nm can be generated by 300~500mW pump at 1064 nm.
- >1W CW at 780 nm can be generated by 3~5W pump at 1560 nm.
(b) Efficient parametric amplification (OPA) and parametric generation (OPG):
- >20dB OPA parametric gain for 1560nm signal can possibly be obtained with CW or Quasi-CW pump power of 10W at 780 nm.
- OPG threshold (equivalent to OPA gain ~80~90dB) can possibly be reached pulsed peak power <1kW at pump of 1064 nm.
(c) Entangled photon pairs generation in Quantum Optics:
- Efficient Type 0 or Type II SPDC has been generated, which can be found in several references.
(d) Infrared (single photon) sensing:
- Quantum Efficiency of up to ~30% can be achieved by MgO:PPLN waveguide based up-conversion process.
(e) Spectrum tailoring for ultrafast or multiple wavelength band conversion:
- Such a broadband or multiple wavelength band conversion has been demonstrated and used in applications which require multiple wavelength band input or fast adjacent wavelength switching between designed wavelength bands for special applications, such as differential absorption or fluorescence applications.
(f) Cascaded Nonlinearity for WDM wavelength band conversion or spectrum inversion for dispersion compensation:
Schematic drawing of dispersion and dispersion compensation of fiber links.
(f-1) Signals transmitted in optical fibers will get distorted after a significant distance due to chromatic dispersion, and two adjacent bits will interfere with each other.
(f-2) Mid-span spectral inverter (MSSI) inverts the signal chirp in the middle point of fiber links and results in an undistorted signal at receiver.
(g) Efficient Electro-optics (EO) Modulation:
PPLN waveguide can also be used for efficient electro-optics (EO) modulator, such as Solic filter, as shown in the link: https://www.hcphotonics.com/blog/ppln-solc-filter
Beyond
With its unique capabilities for engineering phase-matching condition within nonlinear materials, Quasi-Phase Matching (QPM) enables the possibilities to access photon’s full dimensions (wavelength dimension from UV to mid-IR/THz, time dimension from CW to ps/fs/as, and energy dimension from single photon to very huge photon numbers (Watts & up)) for selected nonlinear materials (such as PPLN). As illustrated in figure 6, such a full-spectrum capability of PPLN, along with the advance of other complementary photonics technologies (DPSS-diode pump solid state lasers and fiber lasers; sensor and spectrometer; other wavelength management technologies, etc.), will make several critical contributions to a variety of important applications, such as
- In advanced R&D labs: as an efficient & specialized full-spectrum laser sources (UV/visible to mid-IR/THz) to empower new photonics applications
- In quantum optics: for entangled photon generation and single photon sensing
- In gas spectroscopy: as a narrow-linewidth broad-tunable laser source for gas spectroscopy applications.
- In fluorescence spectroscopy: as a ps/fs/as ultrafast fiber laser source with special wavelengths for pump-probe spectroscopy, two-photon spectroscopy, time-resolved spectroscopy, etc.
- In photo-acoustic imaging/tomography or LIDAR: as a powerful ns Q-switch DPSS laser source with special wavelengths for photo-acoustic imaging/photo-acoustic tomography or LIDAR applications.
- Your innovation !
Figure 6: small photon’s (a) full spectrum dimensions and (b) potential BIG impacts.
© 2017 HC Photonics. All Rights Reserved.